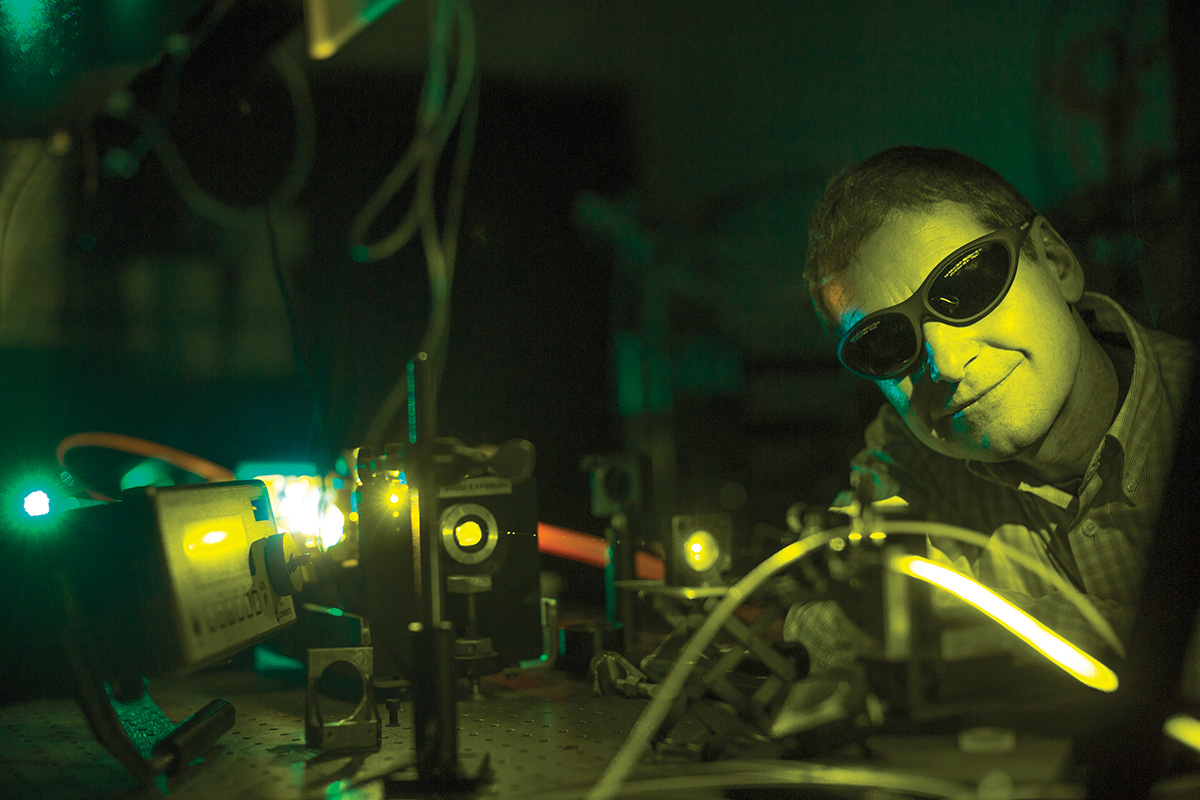
Volkmar Dierolf prefers thinking about defects over perfection—not the defects that lead to degraded performance or reduction of quality, but rather, Dierolf is interested in defects that can be used as tools to enhance a material’s functionality.
Gemstones are a good example of how defects in an otherwise perfect crystal can add beauty. The characteristic color of a ruby comes from the presence of a chromium ion in the aluminum oxide crystal. A different defect in the same material leads to a different color.
Dierolf, AGF University Distinguished Professor and chair of physics, deals in defect engineering. He intentionally laces materials with foreign atoms to enhance their functionality. This type of precise control of atomic or electronic doping turns pure silicon into a material that can be highly functionalized and is the key for devices such as computers and smartphones.
“In my world, defects make the world beautiful,” he says.
Dierolf’s specialty, optical spectroscopy and microscopy of insulating and semiconducting materials, provides the tools he uses to study the physics of incorporating defects in a variety of materials related to light sources, such as lasers and light-emitting diodes (LEDs). He uses his observations to learn how the combination of more than one defect in a material may improve its functionality. One of Dierolf’s goals is to dope semiconductor material used in LEDs to create more efficient white light devices. He is collaborating with a scientist in Japan who produces the Europium-based LED devices that are designed on the basis of the findings obtained in Dierolf’s research group.
Dierolf believes scientists will eventually create LED-based intelligent lighting systems capable of many simultaneous functions, including natural daylight, environmental sensing, humidity control or disinfection. Such advancements must be efficient and energy-saving as well, he says.
“If we can reduce the amount of energy it takes to create lighting, it would make a big impact. In one scheme we envision, you can choose any color for your light fixture. Or you could have an LED display that acts as a skylight window with natural-looking light. Especially, if we could design LEDs to emit the red part of the visible spectrum more efficiently, it would recreate a nice warm feeling without producing wasteful heat, the way incandescents do, but you could also change the color composition to a cooler tone that is better while doing some work.”
Seeing the Light
Dierolf’s team uses near-field optical microscopes and scanning electron microscopes to examine the internal atomic structure of materials. A wealth of data can be found at resolutions down to 50 nanometers, he says.
“If you look deep enough, you can find all kinds of fascinating properties that can not only provide information about the characteristics of a material, but can be used as a tool to shape that material into a useful device that typically is very small and requires control on that length scale.”
Current white LED assemblies are basically on a cap on top of a gallium indium nitride semiconductor with layers of different gallium and indium ratios and additional doping to induce the desired conductivity. The device itself releases photons in the blue spectral region that are then converted in the cap layer into the visible light we see in lamps, on street signals and on high-definition televisions. Depending on the type of cap, the color can be cool, soft or warm white.
Yet, LED fixtures pose challenges. They use far less energy and last longer than standard incandescent bulbs, but the typical approach to constructing white LEDs is less efficient than it could be. Also, they appear imperfect when viewed from different angles and at various brightness levels.
“It is desirable to create the white light within the semiconductor itself and not convert it externally,” says Dierolf.
The standard gallium nitride semiconductor design starts with a base sapphire substrate, onto which crystalline layers of gallium nitride and indium gallium are deposited. This comes with challenges of its own. If the sapphire and gallium or indium gallium nitride lattices have a size mismatch, the ions must be forced into the correct configuration. This creates a strain, which can cause problems. These problems become most severe if a higher indium content is needed to obtain the colors red and green.
The goal of highly efficient white light LED would best be achieved with well-designed red, green and blue LEDs based on nitride materials. However, currently green and red LEDs are currently inefficient.
To overcome the challenges, Dierolf collaborates with groups at Lehigh and in Japan. Collaborating with Nelson Tansu’s group in Lehigh’s PC Rossin College of Engineering and Applied Science, Dierolf’s group studied ways to improve the efficiency of green LEDs through improved semiconductor design. This work resulted in a 2011 paper, “Approaches for high internal quantum efficiency green InGaN light-emitting diodes with large overlap quantum wells,” in the journal Energy Express. Co-authored with Tansu, associate professor of electrical and computer engineering, and two scientists at other institutions, it has been cited almost 300 times and was recognized as one of the five most cited papers among scholarly physics publications from May to June 2013.
To improve the performance of red LEDs, Dierolf wants to put that europium dopant inside the semiconductor itself, a neater, more integrated solution compared to the current approach where such ions are part of the color converting cap.
“We want to transfer the energy that the gallium nitride typically emits into an excitation of the europium atom, which would then emit a red light. The crux of the problem is how to transfer the energy with 100 percent efficiency,” he says.
Precision optical spectroscopy and microscopy allow his team to see a detailed map of the electromagnetic spectrum produced when charged carriers or tunable lasers excite the atoms within semiconductor samples. Changing the environment around the europium ion yields differences in the spectrum and, more importantly, influences the efficiency of excitation.
Dierolf is known for not shying away from the painstaking work of considering small defects. The detailed spatial and spectral maps of the portion of the spectrum they are analyzing may look identical, but a deeper probe often reveals information other researchers may overlook.
“The energy at which the ion is excited is like a distinct fingerprint. That way, we can get a fingerprint of a particular defect and its modifications. If you look carefully and do your measurements systematically, you can get significant information from looking at a small subset of the data area as well as looking at the overall picture,” he says.
In one experiment, he and his team of graduate students typically perform 700 to 1,000 spectral snapshots in two hours. They compare the sample for any minute changes in data. Dierolf uses the emissions data map as a guide to identify and design particular defects.
Lehigh is unique because his team has optimized its own near-field optical microscopes to examine the sample at the nanometer level and analyze particular specks of the emitted spectrum. Electron microscopes are useful because electron beams can focus on small spaces. His group has built an instrument that combines the capability to excite the sample with both a laser and an electron and collects the resulting emission with an optical microscope.
“This can be tedious work,” Dierolf admits. Fortunately, the imaging and analysis are all computer-controlled. Students write programs to evaluate stacks of data.
Defects Create the Perfect Fit
Dierolf, who joined Lehigh in 2000 as the university’s Optical Center for Technology was starting, found an ideal research fit. He earned a Ph.D. in physics from the University of Utah and a habilitation from the University of Paderborn, Germany, which is a qualification earned after submitting an original, independent thesis following a Ph.D. His habilitation was published as a book, Electronic Defect States in Alkali Halides: Effects of Interaction with Molecular Ions, a tome that placed Dierolf as a leader on analyzing materials for defects and combining defects for special uses.
As department chair, he tries to raise Lehigh’s profile in physics, optics and opto-electronics through workshops and programs, including weekly colloquia that bring together leading scholars. He collaborates with other experts internationally. Beyond his research and teaching, Dierolf is now also the co-director of the lauded physics REU program, a competitive summer research program open to undergraduate students across the country who are interested in research experience.
“Certainly, we have world-class research going on at Lehigh,” he says. “Lighting is a billion-dollar industry and a worldwide research endeavor that needs international cooperation to develop ideas. I’m sure the lighting we have will go well beyond what we know now. We’re always enslaved at any one time by what’s technologically possible to build, but we've come a long way from the candle,” he says.
Dierolf also takes his research interests home. He is very concerned about energy-related problems facing the planet and believes solar energy is a promising fuel source that could power the world for the foreseeable future. He has built his own solar-powered systems and uses commercial solar panels on his roof.
“I make my own experiments at home, like how to power batteries so they function more efficiently,” he says. “In my garden are my LED lights, for which I built the control systems myself. I have a strong interest in cleaner energy systems, so I’m using home as a lab to see what can be done in reality, versus what’s just a dream.”